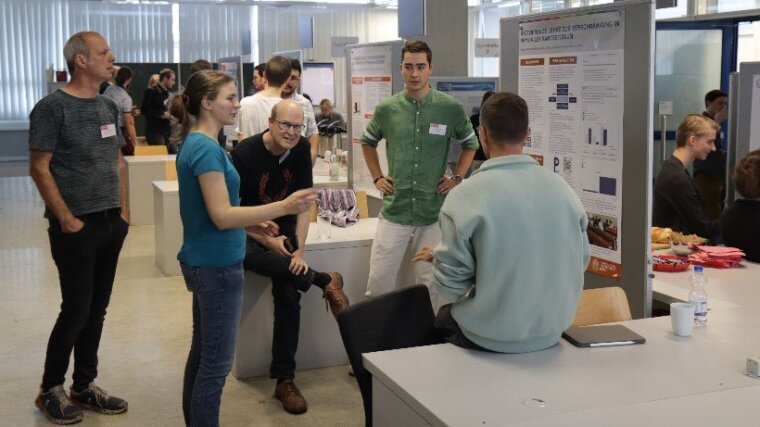
Currently running innovation projects
Since 2023, the following QPhoton Innovation Projects were launched after an external and internal peer-review process:
HoloQ - Developing novel holographic methods for applications with ultracold atoms (Start 07/2024)
Illustration of the principle of computer-generated holograms based on optical fiber tips.
Picture: Falk Eilenberger, Uni Jena.Falk Eilenberger (Jena), Harald Giessen Stuttgart), Johannes Hecker-Denschlag (Ulm)
In this project, we will develop powerful, novel methods based on holography, for applications with ultracold atoms in optical lattices. Optical lattices are ideally suited for a range of important applications such as quantum computing and simulation, and studies of condensed matter physics problems. For such devices it is crucial to precisely determine how many atoms there are at each lattice site and which spin state they have, while only disturbing them minimally. Furthermore, it can be crucial that the optical lattices have a special non-trivial spatial layout in 3D (e.g., not periodic). We must also manipulate lattice sites individually via optical light fields.
These challenges can be elegantly tackled using holographic methods in combination with standard imaging methods. For this, we will develop holographic imaging in an optical lattice with single site and single atom resolution, using only a few photons per atoms. Furthermore, we will develop computer-generated holograms on optical fiber tips to generate non-standard 3D optical light fields in order to trap and manipulate atoms in complex 3D-configurations.
The groups from Jena, Stuttgart, and Ulm each contribute with their respective expertise which is crucial for the success of the project. This includes precision optical design and theoretical holography, microscopic 3D hologram fabrication, and experiments with ultracold atoms.
Levitated photonic microrotors in the quantum regime (Start 07/2024)
Schematic representation of optically controlling the rotational and translational motion of photonic micro-rotors, whose size is comparable to the laser wavelength, and which exhibit Mie-like optical resonances.
Image: Pertsch research group and collaborators.Thomas Pertsch (Jena), Benjamin Stickler (Stuttgart), Sungkun Hong (Ulm)
Tweezer-levitated particles in vacuum offer a unique platform for probing and exploiting quantum aspects of massive mechanical objects. A fascinating prospect is the possibility of using the levitated particle for studying quantum physics on large scales and for developing acceleration and rotation sensors with quantum-limited precision. The field of levitated quantum optomechanics has shown significant progress over the past few years. However, current research has been largely limited to particles with sizes far less than the optical wavelength. To improve existing sensing capabilities and to push quantum experiments to the macroscale, it is desirable to scale up the size and tailor the shape of the particle, which allows for utilizing rich light-matter interactions only available for photonic objects, whose size is comparable to the optical wavelength, and which may thus exhibit Mie-like optical resonances.
In this project, we aim to expand the scope of levitated quantum optomechanics to photonic aspherical particles, whose shape and size is beyond the Rayleigh regime and whose optical response is thus not that of a point dipole. Specifically, we will focus on developing and gaining full quantum motion control over a photonic microrotor, a micron-scale high-aspect-ratio particle with tailored optical and mechanical properties. The successful completion of the proposed project will allow us to achieve quantum-limited control of photonic microrotors, including the cooling of translational and rotational degrees of freedom to the quantum regime. This will pave the way for testing quantum physics in unexplored parameter regimes and for developing levitated gyroscopes as quantum-limited acceleration and rotation sensors with unprecedented controllability.
Integrating atomic vapors for quantum optics on the lithium niobate on insulator platform (Start 06/2023)
Schematic of atomic vapors surrounding a nanostructured integrated waveguide.
Image: Robert Löw, University of Stuttgart.Sina Saravi (Jena), Tilman Pfau (Stuttgart), Joachim Ankerhold (Ulm)
To date, many different technology and solid-state material platforms for integrated optics have been evaluated for large-scale implementation of optical quantum information processing (QIP). However, all such platforms suffer from the same fundamental shortcoming, namely that single photons hardly interact with each other, and that very strong optical nonlinearities are required to induce all-optical interactions. The nonlinearity in solid-state materials is too weak for this purpose, and even the best state-of-the-art nanostructured nonlinear resonators can barely approach this regime. This is the main reason for the lack of on-demand and reproducible sources of single photons and scalable non-Gaussian states of light based on all-optical approaches, where such sources are highly desired for discrete variable and continuous variable QIP. The goal of this project is to create a hybrid platform for integrated optical QIP that can overcome this fundamental shortcoming. This will be done by utilizing the unique properties of atomic vapors of rubidium, in particular their few-photon nonlinearity, integrated with the nanostructured optical platform of lithium niobate on insulator (LNOI), which is one of the most promising candidates for the realization of integrated optical QIP systems. To create this hybrid platform and to explore its unique capabilities, we will combine the complementary expertise of the universities of Jena (expertise on nanostructured LNOI for integrated optics), Ulm (expertise on description of complex quantum systems), and Stuttgart (expertise on atomic vapors for quantum optics). We will establish the practical and technological foundation for the creation of this new hybrid platform and perform preliminary experimental demonstrations showing that this is a functional quantum optical system. In addition, we want to theoretically study the new possibilities for QIP that can result from such a hybrid platform.
NV-qubit for precise sensing of rotational and translational mobilities of active proteins (Start 06/2023)
Symbolic picture of an NV-qubit for sensing of rotational and translational mobilities of active proteins
Picture: Börsch research groupMichael Börsch (Jena), Jörg Wrachtrup (Stuttgart), Fedor Jelezko (Ulm) und Anke Krüger (Stuttgart)
Active proteins control cellular life. The enzyme FoF1-ATP synthase supplies the energy in the form of the ATP molecule, in bacteria, in plants and animals, and in humans. As a rotary nanomotor, this enzyme converts an electrochemical membrane potential into a high-energy chemical bond in ATP in a highly efficient and rapid manner. This stored energy is then used for cellular processes. The complex random dynamics of the three-step motor function can only be observed under the microscope in a spatiotemporally separated, single enzyme. Quantum sensors such as the NV color center in nanodiamonds can detect three-dimensional motion with the highest precision when suitably attached to active proteins as markers.
In this CZS Center QPhoton Innovation project, the expertise in the production of NV-doped nanodiamonds and the measurement and analysis methods of NV spin manipulation are combined with a sensitive but rigid coupling chemistry of solids to soft proteins in aqueous solution. The rotary motor of the purified, modified FoF1-ATP synthase thus drives the tethered sensor, and the NV quantum sensor reports on all individual steps of the motor. Twenty years after the first joint preliminary considerations for this project, all experimental requirements are now being met, and the FoF1-ATP synthase (Jena), the coupling chemistry (Stuttgart), the quantum sensor module (Stuttgart) and the NV spin manipulation tools are being used at the three locations (Ulm) prepared for collaborative quantum-based research of single active proteins at work.
Infrared single-photon detection via superconducting nanowires tailored by disorder, temperature, and magnetic field (Start 04/2023)
Heidemarie Krüger (Jena), Marc Scheffler (Stuttgart), Martin Dressel (Stuttgart), Ciprian Padurariu (Ulm)
Ultrafast superconducting single-photon nanowire detectors (SNSPDs) are versatile detectors with possible applications in quantum sensing, imaging, and information processing, e.g. in the telecom wavelength near 1550 nm in the infrared (IR). Unfortunately, SNSPDs are substantially less efficient in the IR than for visible and higher energy photons. In the project “Infrared single-photon detection via superconducting nanowires tailored by disorder, temperature, and magnetic field” groups from ACP Jena (SNSPD fabrication, transport properties), IQST Stuttgart (dynamics of superconductors), and IQST Ulm (microscopic model) will develop novel design rules and operation to enhance the IR sensitivity of SNSPDs. The research question at the heart of the project is: which new phenomena occur in the interplay of single photon absorption and vortex dynamics in a SNSPD with disorder if an external magnetic field is applied?
Illustration of the superconducting nanowire showing the vortex-lattice interacting with the vortex-antivortex pair generated by an incident photon.
Image: Research Group of Prof. Heidemarie Krüger.